The world’s most powerful neutron microscope is being built in Lund. A team of nuclear researchers from DTU Nutech has contributed to improving the core of the future plant by a factor of 2.5.
In a field outside Lund in southern Sweden, the world’s most powerful neutron source—European Spallation Source (ESS)—is being constructed. The plant is a form of microscope, where researchers by means of neutron radiation can ‘see’ into biological materials and metals to get a detailed picture of how they are constructed at atomic level.
At the heart of the ESS is a plant that generates neutrons by accelerating particles at high speed and firing them into the heavy metal tungsten, which emits neutrons—a process known as a spallation. The neutrons are then slowed down in a so-called moderator, after which they can be fired out in a ‘beamline’. Each beamline has its own special instrument, depending on the type of experiments to be carried out on the line. Here, the neutrons strike the material and—regardless of whether it is soft, such as a biological sample, or hard, such as metal—penetrate deep into the material without damaging it.
Working with neutrons, however, is not as straightforward as it sounds, explains nuclear physicist and ESS Neutronics Group Leader Luca Zanini:
“Neutrons have no charge and therefore cannot be controlled in the same way as charged particles. Imagine that out of a million neutrons created in the spallation process you end up perhaps with only
one neutron where the researchers want it.”
You take a proton..
In the ESS core chamber sits a round disk with a diameter of approximately 2.5 metres. The disk is made of the extremely hard element tungsten. When you fire protons at tungsten at a speed approaching the speed of light, particles of the core evaporate, releasing the neutrons which can subsequently be used to study material samples. Above the tungsten disk sits the so-called moderator made of liquid hydrogen—an extremely cold substance whose minus 253 degrees Celsius cools the wild neutrons, denying them of speed and energy so that they can be guided on to experimental set-ups. The tungsten disk and moderator are surrounded by the element Beryllium, which is especially well-suited for reflecting neutrons. Thus, many of the neutrons which would otherwise be lost are reflected back into the moderator.
However, as a general rule the neutrons are spread in all directions, and the challenge, therefore, is to maximize the number of neutrons that can be released to the surrounding 22 beamlines. A group of researchers, including PhD student Troels Schönfeldt and postdoc Esben Klinkby from DTU Nutech, has decided to address this very challenge.
"Sometimes you get a good idea and can take a major step forward. And that’s what has happened here."
Troels Schönfeldt, PhD student at DTU Nutech
Together with the Neutronics group in Lund, DTU Nutech researchers have made radical changes in both the design and location of the moderator.
“The first step was gaining a deeper understanding of what is happening in the actual process and then adjusting the various parameters with simulation tools to optimize the result. Sometimes you get a good idea and can take a major step forward. And that’s what has happened here,” explains Troels Schönfeldt, who continues:
“Typically, a moderator resembles a bucket with liquid hydrogen or methane located next to a water tub. However, through computer simulations we have shown that a flat moderator generates many more useful neutrons for the experiments. This discovery led us to a new and innovative design, which we call the butterfly, where the water moderator is located centrally between two flat blades with liquid hydrogen. This gives us approximately 2.5 times more neutrons than expected.”
This is a significant improvement of the ESS core itself—a fact that greatly pleases ESS’ Luca Zanini:
“Through history it has proved extremely complicated to increase the number of neutrons. It is therefore truly impressive that DTU researchers can increase the neutron output by no less than a factor of 2.5 with the new design,” he says.
The entire ESS facility, which is expected to be completed by 2019, will cost close to EUR 2bn.
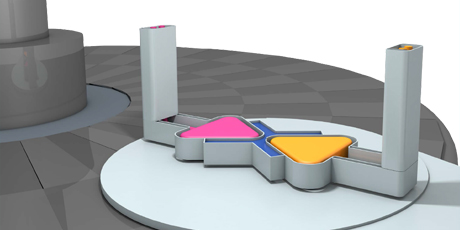
|
|
The equipment in the illustration constitutes the small core of approximately 2.5 metres in diameter at the centre of the huge spallation facility in Lund.
|
Article in DYNAMO no. 42, DTU's quarterly magazine in Danish.
- Researchers from DTU Nutech have radically altered the moderator’s design from a barrel-shaped unit to two flat parts which together form a characteristic butterfly shape. The design increases the number of neutrons transported out into beamlines by a factor of 2.5.
- The moderator is placed in a water bath at room temperature, which in itself reduces the speed of the neutrons. Neutrons passing the bath are called thermal neutrons and are used either alone or together with the cold neutrons from the moderator by different research groups whose research ranges from everything from fundamental physics, chemistry, and biotechnology to energy materials and archaeology.
- The water layer area around the moderator has been increased so that more of the fast neutrons are captured and become thermal.
- There will be 22 beamlines leading cold or thermal neutrons to the researchers’ experimental set-ups. Depending on the experiment to be carried out, the researchers can decide whether to use cold neutrons, thermal neutrons or a combination of the two.
- The target for the proton beam is the tungsten disk, which is divided into thin pieces resembling a layer cake to avoid overheating. The disk rotates, so that a new piece is constantly exposed to the powerful proton beam.